Atoms in ancient minerals tell a smashing history
Every planet has its secrets, especially the events of its youth billions of years ago. However, patterns of atoms in tiny fragments of ancient crystals can reveal some of those secrets – the date of events such as giant meteorite impacts. Researchers from Western University and the University of Portsmouth made the discovery using the 150-km (93-mile) wide Sudbury crater as a test site.
Shock waves from the impact of the meteorite that formed the Sudbury structure almost two billion years ago also deformed the minerals that make up the rocks beneath the crater. Some of the smallest crystals (like the
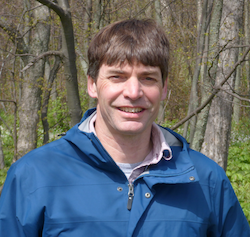
“These can be used as tiny clocks that are the basis for our geologic time scale,” says
New techniques can now take a microscopic crystal fragment and measure the age of the rock, the timing of crater formation and even later crater warping in a mountain-building event. The work is thanks to an international team using
“Using the atom probe to go from the rock to the crystal to its atomic level is like zooming in with the ultimate Google Earth,” Moser says.
“Microminerals, although less than the width of a human hair, are the ultimate ‘survivor crystals,’” he continues. “These can reveal information on the relative age and nature of processes that shaped our environment, from the precise timing of a modern volcanic eruption to the establishment of water on a planet four billion years ago.”
Moser points out that the force of a meteorite collision can be “Earth-sterilizing,” in that it melts the surface of the planet and causes oceans to evaporate.
“Some meteorite crashes are so large, they reshape the entire planet, as happened with Mars,” Moser adds. “Our research allows us to determine when these impacts occurred, and when they were so intense that life forms would not survive. Finding the point where the effects stop is like setting a stopwatch for the start of early life forms.”
That timing is central to understanding the formation and evolution of planetary crusts and the habitability of planets.
Resetting the clock
The main information about time comes from the radioactive decay process.
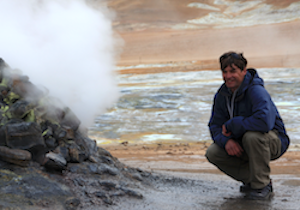
“All minerals we look at have tiny amounts of uranium when they form, but none of the ‘daughter’ product,” Moser explains. “Over time, the uranium changes into daughter lead. In large, high-energy impact events, you can remove some of that lead and basically reset the clock in the mineral to find when this high-energy impact occurred.”
Conventional techniques involve destroying and mixing most of a grain to measure the uranium and lead. However, damaged domains in the mineral can allow some of the lead to move out, resulting in a false “mixed” date that doesn’t correspond to a real event.
The new atom probe tomography technique combines special equipment at the ZAPLab and CAMECA Laboratories in the U.S. to go in at the microscopic level. The instrument can then slice and lift out tiny pieces of baddeleyite, which is common in terrestrial, Martian and lunar rocks and meteorites.
Shock waves from meteorite collisions will twist and
The team’s findings, published in the journal Nature Communications, reveal domains of different uranium-to-lead age that match the history of the crust of northern Ontario. They also show fascinating clustering of impurities into ripples, possibly created by the shock wave.
“This atomic-scale approach holds great potential in establishing a more accurate chronology of the formation and evolution of planetary crusts,” Moser says. “Plus, the amount taken is so tiny, it hardly affects the sample. That’s especially important for rare and valuable samples like meteorites.”
Understanding the memory cells of the earth
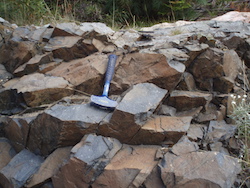
The outcrop at the Sudbury crater, where Moser’s team obtained crystals of baddeleyite.
Moser’s fascination with planetary science goes back to growing up in southern Ontario, searching the night skies with his telescope. He became interested in giant meteorite impact structures more than 20 years ago when visiting the largest such structure on Earth in South Africa (Sudbury is the second largest), and has active research projects investigating them in the Americas, Africa and Europe.
“If we can discover what the impacts do to minerals at
Moser feels the research opens up a new world for studying planetary history.
“I hope this will give a better understanding of the initial conditions on our planet that led to the world we have today,” he adds. “Our planet is a product of billions of years of stories, and understanding the memory cells of the earth helps us appreciate where we are in the immense history of our solar system.”